mirror of
https://github.com/llvm/llvm-project.git
synced 2025-04-28 14:06:08 +00:00
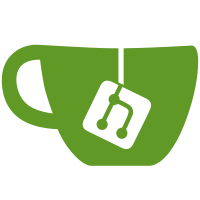
In general, if we see an allocation, we associate the immutable memory space with the constructed memory region. This works fine if we see the allocation. However, with symbolic regions it's not great because there we don't know anything about their memory spaces, thus put them into the Unknown space. The unfortunate consequence is that once we learn about some aliasing with this Symbolic Region, we can't change the memory space to the deduced one. In this patch, we open up the memory spaces as a trait, basically allowing associating a better memory space with a memregion that was created with the Unknown memory space. As a side effect, this means that now queriing the memory space of a region depends on the State, but many places in the analyzer, such as the Store, doesn't have (and cannot have) access to the State by design. This means that some uses must solely rely on the memspaces of the region, but any other users should use the getter taking a State. Co-authored-by: Balazs Benics <benicsbalazs@gmail.com>
1433 lines
54 KiB
C++
1433 lines
54 KiB
C++
// SimpleSValBuilder.cpp - A basic SValBuilder -----------------------*- C++ -*-
|
|
//
|
|
// Part of the LLVM Project, under the Apache License v2.0 with LLVM Exceptions.
|
|
// See https://llvm.org/LICENSE.txt for license information.
|
|
// SPDX-License-Identifier: Apache-2.0 WITH LLVM-exception
|
|
//
|
|
//===----------------------------------------------------------------------===//
|
|
//
|
|
// This file defines SimpleSValBuilder, a basic implementation of SValBuilder.
|
|
//
|
|
//===----------------------------------------------------------------------===//
|
|
|
|
#include "clang/StaticAnalyzer/Core/PathSensitive/APSIntPtr.h"
|
|
#include "clang/StaticAnalyzer/Core/PathSensitive/APSIntType.h"
|
|
#include "clang/StaticAnalyzer/Core/PathSensitive/ExprEngine.h"
|
|
#include "clang/StaticAnalyzer/Core/PathSensitive/ProgramState.h"
|
|
#include "clang/StaticAnalyzer/Core/PathSensitive/SValBuilder.h"
|
|
#include "clang/StaticAnalyzer/Core/PathSensitive/SValVisitor.h"
|
|
#include <optional>
|
|
|
|
using namespace clang;
|
|
using namespace ento;
|
|
|
|
namespace {
|
|
class SimpleSValBuilder : public SValBuilder {
|
|
|
|
// Query the constraint manager whether the SVal has only one possible
|
|
// (integer) value. If that is the case, the value is returned. Otherwise,
|
|
// returns NULL.
|
|
// This is an implementation detail. Checkers should use `getKnownValue()`
|
|
// instead.
|
|
static const llvm::APSInt *getConstValue(ProgramStateRef state, SVal V);
|
|
|
|
// Helper function that returns the value stored in a nonloc::ConcreteInt or
|
|
// loc::ConcreteInt.
|
|
static const llvm::APSInt *getConcreteValue(SVal V);
|
|
|
|
// With one `simplifySValOnce` call, a compound symbols might collapse to
|
|
// simpler symbol tree that is still possible to further simplify. Thus, we
|
|
// do the simplification on a new symbol tree until we reach the simplest
|
|
// form, i.e. the fixpoint.
|
|
// Consider the following symbol `(b * b) * b * b` which has this tree:
|
|
// *
|
|
// / \
|
|
// * b
|
|
// / \
|
|
// / b
|
|
// (b * b)
|
|
// Now, if the `b * b == 1` new constraint is added then during the first
|
|
// iteration we have the following transformations:
|
|
// * *
|
|
// / \ / \
|
|
// * b --> b b
|
|
// / \
|
|
// / b
|
|
// 1
|
|
// We need another iteration to reach the final result `1`.
|
|
SVal simplifyUntilFixpoint(ProgramStateRef State, SVal Val);
|
|
|
|
// Recursively descends into symbolic expressions and replaces symbols
|
|
// with their known values (in the sense of the getConstValue() method).
|
|
// We traverse the symbol tree and query the constraint values for the
|
|
// sub-trees and if a value is a constant we do the constant folding.
|
|
SVal simplifySValOnce(ProgramStateRef State, SVal V);
|
|
|
|
public:
|
|
SimpleSValBuilder(llvm::BumpPtrAllocator &alloc, ASTContext &context,
|
|
ProgramStateManager &stateMgr)
|
|
: SValBuilder(alloc, context, stateMgr) {}
|
|
~SimpleSValBuilder() override {}
|
|
|
|
SVal evalBinOpNN(ProgramStateRef state, BinaryOperator::Opcode op,
|
|
NonLoc lhs, NonLoc rhs, QualType resultTy) override;
|
|
SVal evalBinOpLL(ProgramStateRef state, BinaryOperator::Opcode op,
|
|
Loc lhs, Loc rhs, QualType resultTy) override;
|
|
SVal evalBinOpLN(ProgramStateRef state, BinaryOperator::Opcode op,
|
|
Loc lhs, NonLoc rhs, QualType resultTy) override;
|
|
|
|
/// Evaluates a given SVal by recursively evaluating and
|
|
/// simplifying the children SVals. If the SVal has only one possible
|
|
/// (integer) value, that value is returned. Otherwise, returns NULL.
|
|
const llvm::APSInt *getKnownValue(ProgramStateRef state, SVal V) override;
|
|
|
|
/// Evaluates a given SVal by recursively evaluating and simplifying the
|
|
/// children SVals, then returns its minimal possible (integer) value. If the
|
|
/// constraint manager cannot provide a meaningful answer, this returns NULL.
|
|
const llvm::APSInt *getMinValue(ProgramStateRef state, SVal V) override;
|
|
|
|
/// Evaluates a given SVal by recursively evaluating and simplifying the
|
|
/// children SVals, then returns its maximal possible (integer) value. If the
|
|
/// constraint manager cannot provide a meaningful answer, this returns NULL.
|
|
const llvm::APSInt *getMaxValue(ProgramStateRef state, SVal V) override;
|
|
|
|
SVal simplifySVal(ProgramStateRef State, SVal V) override;
|
|
|
|
SVal MakeSymIntVal(const SymExpr *LHS, BinaryOperator::Opcode op,
|
|
const llvm::APSInt &RHS, QualType resultTy);
|
|
};
|
|
} // end anonymous namespace
|
|
|
|
SValBuilder *ento::createSimpleSValBuilder(llvm::BumpPtrAllocator &alloc,
|
|
ASTContext &context,
|
|
ProgramStateManager &stateMgr) {
|
|
return new SimpleSValBuilder(alloc, context, stateMgr);
|
|
}
|
|
|
|
// Checks if the negation the value and flipping sign preserve
|
|
// the semantics on the operation in the resultType
|
|
static bool isNegationValuePreserving(const llvm::APSInt &Value,
|
|
APSIntType ResultType) {
|
|
const unsigned ValueBits = Value.getSignificantBits();
|
|
if (ValueBits == ResultType.getBitWidth()) {
|
|
// The value is the lowest negative value that is representable
|
|
// in signed integer with bitWith of result type. The
|
|
// negation is representable if resultType is unsigned.
|
|
return ResultType.isUnsigned();
|
|
}
|
|
|
|
// If resultType bitWith is higher that number of bits required
|
|
// to represent RHS, the sign flip produce same value.
|
|
return ValueBits < ResultType.getBitWidth();
|
|
}
|
|
|
|
//===----------------------------------------------------------------------===//
|
|
// Transfer function for binary operators.
|
|
//===----------------------------------------------------------------------===//
|
|
|
|
SVal SimpleSValBuilder::MakeSymIntVal(const SymExpr *LHS,
|
|
BinaryOperator::Opcode op,
|
|
const llvm::APSInt &RHS,
|
|
QualType resultTy) {
|
|
bool isIdempotent = false;
|
|
|
|
// Check for a few special cases with known reductions first.
|
|
switch (op) {
|
|
default:
|
|
// We can't reduce this case; just treat it normally.
|
|
break;
|
|
case BO_Mul:
|
|
// a*0 and a*1
|
|
if (RHS == 0)
|
|
return makeIntVal(0, resultTy);
|
|
else if (RHS == 1)
|
|
isIdempotent = true;
|
|
break;
|
|
case BO_Div:
|
|
// a/0 and a/1
|
|
if (RHS == 0)
|
|
// This is also handled elsewhere.
|
|
return UndefinedVal();
|
|
else if (RHS == 1)
|
|
isIdempotent = true;
|
|
break;
|
|
case BO_Rem:
|
|
// a%0 and a%1
|
|
if (RHS == 0)
|
|
// This is also handled elsewhere.
|
|
return UndefinedVal();
|
|
else if (RHS == 1)
|
|
return makeIntVal(0, resultTy);
|
|
break;
|
|
case BO_Add:
|
|
case BO_Sub:
|
|
case BO_Shl:
|
|
case BO_Shr:
|
|
case BO_Xor:
|
|
// a+0, a-0, a<<0, a>>0, a^0
|
|
if (RHS == 0)
|
|
isIdempotent = true;
|
|
break;
|
|
case BO_And:
|
|
// a&0 and a&(~0)
|
|
if (RHS == 0)
|
|
return makeIntVal(0, resultTy);
|
|
else if (RHS.isAllOnes())
|
|
isIdempotent = true;
|
|
break;
|
|
case BO_Or:
|
|
// a|0 and a|(~0)
|
|
if (RHS == 0)
|
|
isIdempotent = true;
|
|
else if (RHS.isAllOnes()) {
|
|
return nonloc::ConcreteInt(BasicVals.Convert(resultTy, RHS));
|
|
}
|
|
break;
|
|
}
|
|
|
|
// Idempotent ops (like a*1) can still change the type of an expression.
|
|
// Wrap the LHS up in a NonLoc again and let evalCast do the
|
|
// dirty work.
|
|
if (isIdempotent)
|
|
return evalCast(nonloc::SymbolVal(LHS), resultTy, QualType{});
|
|
|
|
// If we reach this point, the expression cannot be simplified.
|
|
// Make a SymbolVal for the entire expression, after converting the RHS.
|
|
std::optional<APSIntPtr> ConvertedRHS = BasicVals.getValue(RHS);
|
|
if (BinaryOperator::isComparisonOp(op)) {
|
|
// We're looking for a type big enough to compare the symbolic value
|
|
// with the given constant.
|
|
// FIXME: This is an approximation of Sema::UsualArithmeticConversions.
|
|
ASTContext &Ctx = getContext();
|
|
QualType SymbolType = LHS->getType();
|
|
uint64_t ValWidth = RHS.getBitWidth();
|
|
uint64_t TypeWidth = Ctx.getTypeSize(SymbolType);
|
|
|
|
if (ValWidth < TypeWidth) {
|
|
// If the value is too small, extend it.
|
|
ConvertedRHS = BasicVals.Convert(SymbolType, RHS);
|
|
} else if (ValWidth == TypeWidth) {
|
|
// If the value is signed but the symbol is unsigned, do the comparison
|
|
// in unsigned space. [C99 6.3.1.8]
|
|
// (For the opposite case, the value is already unsigned.)
|
|
if (RHS.isSigned() && !SymbolType->isSignedIntegerOrEnumerationType())
|
|
ConvertedRHS = BasicVals.Convert(SymbolType, RHS);
|
|
}
|
|
} else if (BinaryOperator::isAdditiveOp(op) && RHS.isNegative()) {
|
|
// Change a+(-N) into a-N, and a-(-N) into a+N
|
|
// Adjust addition/subtraction of negative value, to
|
|
// subtraction/addition of the negated value.
|
|
APSIntType resultIntTy = BasicVals.getAPSIntType(resultTy);
|
|
if (isNegationValuePreserving(RHS, resultIntTy)) {
|
|
ConvertedRHS = BasicVals.getValue(-resultIntTy.convert(RHS));
|
|
op = (op == BO_Add) ? BO_Sub : BO_Add;
|
|
} else {
|
|
ConvertedRHS = BasicVals.Convert(resultTy, RHS);
|
|
}
|
|
} else
|
|
ConvertedRHS = BasicVals.Convert(resultTy, RHS);
|
|
|
|
return makeNonLoc(LHS, op, *ConvertedRHS, resultTy);
|
|
}
|
|
|
|
// See if Sym is known to be a relation Rel with Bound.
|
|
static bool isInRelation(BinaryOperator::Opcode Rel, SymbolRef Sym,
|
|
llvm::APSInt Bound, ProgramStateRef State) {
|
|
SValBuilder &SVB = State->getStateManager().getSValBuilder();
|
|
BasicValueFactory &BV = SVB.getBasicValueFactory();
|
|
SVal Result = SVB.evalBinOpNN(State, Rel, nonloc::SymbolVal(Sym),
|
|
nonloc::ConcreteInt(BV.getValue(Bound)),
|
|
SVB.getConditionType());
|
|
if (auto DV = Result.getAs<DefinedSVal>()) {
|
|
return !State->assume(*DV, false);
|
|
}
|
|
return false;
|
|
}
|
|
|
|
// See if Sym is known to be within [min/4, max/4], where min and max
|
|
// are the bounds of the symbol's integral type. With such symbols,
|
|
// some manipulations can be performed without the risk of overflow.
|
|
// assume() doesn't cause infinite recursion because we should be dealing
|
|
// with simpler symbols on every recursive call.
|
|
static bool isWithinConstantOverflowBounds(SymbolRef Sym,
|
|
ProgramStateRef State) {
|
|
SValBuilder &SVB = State->getStateManager().getSValBuilder();
|
|
BasicValueFactory &BV = SVB.getBasicValueFactory();
|
|
|
|
QualType T = Sym->getType();
|
|
assert(T->isSignedIntegerOrEnumerationType() &&
|
|
"This only works with signed integers!");
|
|
APSIntType AT = BV.getAPSIntType(T);
|
|
|
|
llvm::APSInt Max = AT.getMaxValue() / AT.getValue(4), Min = -Max;
|
|
return isInRelation(BO_LE, Sym, Max, State) &&
|
|
isInRelation(BO_GE, Sym, Min, State);
|
|
}
|
|
|
|
// Same for the concrete integers: see if I is within [min/4, max/4].
|
|
static bool isWithinConstantOverflowBounds(llvm::APSInt I) {
|
|
APSIntType AT(I);
|
|
assert(!AT.isUnsigned() &&
|
|
"This only works with signed integers!");
|
|
|
|
llvm::APSInt Max = AT.getMaxValue() / AT.getValue(4), Min = -Max;
|
|
return (I <= Max) && (I >= -Max);
|
|
}
|
|
|
|
static std::pair<SymbolRef, APSIntPtr> decomposeSymbol(SymbolRef Sym,
|
|
BasicValueFactory &BV) {
|
|
if (const auto *SymInt = dyn_cast<SymIntExpr>(Sym))
|
|
if (BinaryOperator::isAdditiveOp(SymInt->getOpcode()))
|
|
return std::make_pair(SymInt->getLHS(),
|
|
(SymInt->getOpcode() == BO_Add)
|
|
? BV.getValue(SymInt->getRHS())
|
|
: BV.getValue(-SymInt->getRHS()));
|
|
|
|
// Fail to decompose: "reduce" the problem to the "$x + 0" case.
|
|
return std::make_pair(Sym, BV.getValue(0, Sym->getType()));
|
|
}
|
|
|
|
// Simplify "(LSym + LInt) Op (RSym + RInt)" assuming all values are of the
|
|
// same signed integral type and no overflows occur (which should be checked
|
|
// by the caller).
|
|
static NonLoc doRearrangeUnchecked(ProgramStateRef State,
|
|
BinaryOperator::Opcode Op,
|
|
SymbolRef LSym, llvm::APSInt LInt,
|
|
SymbolRef RSym, llvm::APSInt RInt) {
|
|
SValBuilder &SVB = State->getStateManager().getSValBuilder();
|
|
BasicValueFactory &BV = SVB.getBasicValueFactory();
|
|
SymbolManager &SymMgr = SVB.getSymbolManager();
|
|
|
|
QualType SymTy = LSym->getType();
|
|
assert(SymTy == RSym->getType() &&
|
|
"Symbols are not of the same type!");
|
|
assert(APSIntType(LInt) == BV.getAPSIntType(SymTy) &&
|
|
"Integers are not of the same type as symbols!");
|
|
assert(APSIntType(RInt) == BV.getAPSIntType(SymTy) &&
|
|
"Integers are not of the same type as symbols!");
|
|
|
|
QualType ResultTy;
|
|
if (BinaryOperator::isComparisonOp(Op))
|
|
ResultTy = SVB.getConditionType();
|
|
else if (BinaryOperator::isAdditiveOp(Op))
|
|
ResultTy = SymTy;
|
|
else
|
|
llvm_unreachable("Operation not suitable for unchecked rearrangement!");
|
|
|
|
if (LSym == RSym)
|
|
return SVB
|
|
.evalBinOpNN(State, Op, nonloc::ConcreteInt(BV.getValue(LInt)),
|
|
nonloc::ConcreteInt(BV.getValue(RInt)), ResultTy)
|
|
.castAs<NonLoc>();
|
|
|
|
SymbolRef ResultSym = nullptr;
|
|
BinaryOperator::Opcode ResultOp;
|
|
llvm::APSInt ResultInt;
|
|
if (BinaryOperator::isComparisonOp(Op)) {
|
|
// Prefer comparing to a non-negative number.
|
|
// FIXME: Maybe it'd be better to have consistency in
|
|
// "$x - $y" vs. "$y - $x" because those are solver's keys.
|
|
if (LInt > RInt) {
|
|
ResultSym = SymMgr.acquire<SymSymExpr>(RSym, BO_Sub, LSym, SymTy);
|
|
ResultOp = BinaryOperator::reverseComparisonOp(Op);
|
|
ResultInt = LInt - RInt; // Opposite order!
|
|
} else {
|
|
ResultSym = SymMgr.acquire<SymSymExpr>(LSym, BO_Sub, RSym, SymTy);
|
|
ResultOp = Op;
|
|
ResultInt = RInt - LInt; // Opposite order!
|
|
}
|
|
} else {
|
|
ResultSym = SymMgr.acquire<SymSymExpr>(LSym, Op, RSym, SymTy);
|
|
ResultInt = (Op == BO_Add) ? (LInt + RInt) : (LInt - RInt);
|
|
ResultOp = BO_Add;
|
|
// Bring back the cosmetic difference.
|
|
if (ResultInt < 0) {
|
|
ResultInt = -ResultInt;
|
|
ResultOp = BO_Sub;
|
|
} else if (ResultInt == 0) {
|
|
// Shortcut: Simplify "$x + 0" to "$x".
|
|
return nonloc::SymbolVal(ResultSym);
|
|
}
|
|
}
|
|
APSIntPtr PersistentResultInt = BV.getValue(ResultInt);
|
|
return nonloc::SymbolVal(SymMgr.acquire<SymIntExpr>(
|
|
ResultSym, ResultOp, PersistentResultInt, ResultTy));
|
|
}
|
|
|
|
// Rearrange if symbol type matches the result type and if the operator is a
|
|
// comparison operator, both symbol and constant must be within constant
|
|
// overflow bounds.
|
|
static bool shouldRearrange(ProgramStateRef State, BinaryOperator::Opcode Op,
|
|
SymbolRef Sym, llvm::APSInt Int, QualType Ty) {
|
|
return Sym->getType() == Ty &&
|
|
(!BinaryOperator::isComparisonOp(Op) ||
|
|
(isWithinConstantOverflowBounds(Sym, State) &&
|
|
isWithinConstantOverflowBounds(Int)));
|
|
}
|
|
|
|
static std::optional<NonLoc> tryRearrange(ProgramStateRef State,
|
|
BinaryOperator::Opcode Op, NonLoc Lhs,
|
|
NonLoc Rhs, QualType ResultTy) {
|
|
ProgramStateManager &StateMgr = State->getStateManager();
|
|
SValBuilder &SVB = StateMgr.getSValBuilder();
|
|
|
|
// We expect everything to be of the same type - this type.
|
|
QualType SingleTy;
|
|
|
|
// FIXME: After putting complexity threshold to the symbols we can always
|
|
// rearrange additive operations but rearrange comparisons only if
|
|
// option is set.
|
|
if (!SVB.getAnalyzerOptions().ShouldAggressivelySimplifyBinaryOperation)
|
|
return std::nullopt;
|
|
|
|
SymbolRef LSym = Lhs.getAsSymbol();
|
|
if (!LSym)
|
|
return std::nullopt;
|
|
|
|
if (BinaryOperator::isComparisonOp(Op)) {
|
|
SingleTy = LSym->getType();
|
|
if (ResultTy != SVB.getConditionType())
|
|
return std::nullopt;
|
|
// Initialize SingleTy later with a symbol's type.
|
|
} else if (BinaryOperator::isAdditiveOp(Op)) {
|
|
SingleTy = ResultTy;
|
|
if (LSym->getType() != SingleTy)
|
|
return std::nullopt;
|
|
} else {
|
|
// Don't rearrange other operations.
|
|
return std::nullopt;
|
|
}
|
|
|
|
assert(!SingleTy.isNull() && "We should have figured out the type by now!");
|
|
|
|
// Rearrange signed symbolic expressions only
|
|
if (!SingleTy->isSignedIntegerOrEnumerationType())
|
|
return std::nullopt;
|
|
|
|
SymbolRef RSym = Rhs.getAsSymbol();
|
|
if (!RSym || RSym->getType() != SingleTy)
|
|
return std::nullopt;
|
|
|
|
BasicValueFactory &BV = State->getBasicVals();
|
|
llvm::APSInt LInt, RInt;
|
|
std::tie(LSym, LInt) = decomposeSymbol(LSym, BV);
|
|
std::tie(RSym, RInt) = decomposeSymbol(RSym, BV);
|
|
if (!shouldRearrange(State, Op, LSym, LInt, SingleTy) ||
|
|
!shouldRearrange(State, Op, RSym, RInt, SingleTy))
|
|
return std::nullopt;
|
|
|
|
// We know that no overflows can occur anymore.
|
|
return doRearrangeUnchecked(State, Op, LSym, LInt, RSym, RInt);
|
|
}
|
|
|
|
SVal SimpleSValBuilder::evalBinOpNN(ProgramStateRef state,
|
|
BinaryOperator::Opcode op,
|
|
NonLoc lhs, NonLoc rhs,
|
|
QualType resultTy) {
|
|
NonLoc InputLHS = lhs;
|
|
NonLoc InputRHS = rhs;
|
|
|
|
// Constraints may have changed since the creation of a bound SVal. Check if
|
|
// the values can be simplified based on those new constraints.
|
|
SVal simplifiedLhs = simplifySVal(state, lhs);
|
|
SVal simplifiedRhs = simplifySVal(state, rhs);
|
|
if (auto simplifiedLhsAsNonLoc = simplifiedLhs.getAs<NonLoc>())
|
|
lhs = *simplifiedLhsAsNonLoc;
|
|
if (auto simplifiedRhsAsNonLoc = simplifiedRhs.getAs<NonLoc>())
|
|
rhs = *simplifiedRhsAsNonLoc;
|
|
|
|
// Handle trivial case where left-side and right-side are the same.
|
|
if (lhs == rhs)
|
|
switch (op) {
|
|
default:
|
|
break;
|
|
case BO_EQ:
|
|
case BO_LE:
|
|
case BO_GE:
|
|
return makeTruthVal(true, resultTy);
|
|
case BO_LT:
|
|
case BO_GT:
|
|
case BO_NE:
|
|
return makeTruthVal(false, resultTy);
|
|
case BO_Xor:
|
|
case BO_Sub:
|
|
if (resultTy->isIntegralOrEnumerationType())
|
|
return makeIntVal(0, resultTy);
|
|
return evalCast(makeIntVal(0, /*isUnsigned=*/false), resultTy,
|
|
QualType{});
|
|
case BO_Or:
|
|
case BO_And:
|
|
return evalCast(lhs, resultTy, QualType{});
|
|
}
|
|
|
|
while (true) {
|
|
switch (lhs.getKind()) {
|
|
default:
|
|
return makeSymExprValNN(op, lhs, rhs, resultTy);
|
|
case nonloc::PointerToMemberKind: {
|
|
assert(rhs.getKind() == nonloc::PointerToMemberKind &&
|
|
"Both SVals should have pointer-to-member-type");
|
|
auto LPTM = lhs.castAs<nonloc::PointerToMember>(),
|
|
RPTM = rhs.castAs<nonloc::PointerToMember>();
|
|
auto LPTMD = LPTM.getPTMData(), RPTMD = RPTM.getPTMData();
|
|
switch (op) {
|
|
case BO_EQ:
|
|
return makeTruthVal(LPTMD == RPTMD, resultTy);
|
|
case BO_NE:
|
|
return makeTruthVal(LPTMD != RPTMD, resultTy);
|
|
default:
|
|
return UnknownVal();
|
|
}
|
|
}
|
|
case nonloc::LocAsIntegerKind: {
|
|
Loc lhsL = lhs.castAs<nonloc::LocAsInteger>().getLoc();
|
|
switch (rhs.getKind()) {
|
|
case nonloc::LocAsIntegerKind:
|
|
// FIXME: at the moment the implementation
|
|
// of modeling "pointers as integers" is not complete.
|
|
if (!BinaryOperator::isComparisonOp(op))
|
|
return UnknownVal();
|
|
return evalBinOpLL(state, op, lhsL,
|
|
rhs.castAs<nonloc::LocAsInteger>().getLoc(),
|
|
resultTy);
|
|
case nonloc::ConcreteIntKind: {
|
|
// FIXME: at the moment the implementation
|
|
// of modeling "pointers as integers" is not complete.
|
|
if (!BinaryOperator::isComparisonOp(op))
|
|
return UnknownVal();
|
|
// Transform the integer into a location and compare.
|
|
// FIXME: This only makes sense for comparisons. If we want to, say,
|
|
// add 1 to a LocAsInteger, we'd better unpack the Loc and add to it,
|
|
// then pack it back into a LocAsInteger.
|
|
llvm::APSInt i = rhs.castAs<nonloc::ConcreteInt>().getValue();
|
|
// If the region has a symbolic base, pay attention to the type; it
|
|
// might be coming from a non-default address space. For non-symbolic
|
|
// regions it doesn't matter that much because such comparisons would
|
|
// most likely evaluate to concrete false anyway. FIXME: We might
|
|
// still need to handle the non-comparison case.
|
|
if (SymbolRef lSym = lhs.getAsLocSymbol(true))
|
|
BasicVals.getAPSIntType(lSym->getType()).apply(i);
|
|
else
|
|
BasicVals.getAPSIntType(Context.VoidPtrTy).apply(i);
|
|
return evalBinOpLL(state, op, lhsL, makeLoc(i), resultTy);
|
|
}
|
|
default:
|
|
switch (op) {
|
|
case BO_EQ:
|
|
return makeTruthVal(false, resultTy);
|
|
case BO_NE:
|
|
return makeTruthVal(true, resultTy);
|
|
default:
|
|
// This case also handles pointer arithmetic.
|
|
return makeSymExprValNN(op, InputLHS, InputRHS, resultTy);
|
|
}
|
|
}
|
|
}
|
|
case nonloc::ConcreteIntKind: {
|
|
llvm::APSInt LHSValue = lhs.castAs<nonloc::ConcreteInt>().getValue();
|
|
|
|
// If we're dealing with two known constants, just perform the operation.
|
|
if (const llvm::APSInt *KnownRHSValue = getConstValue(state, rhs)) {
|
|
llvm::APSInt RHSValue = *KnownRHSValue;
|
|
if (BinaryOperator::isComparisonOp(op)) {
|
|
// We're looking for a type big enough to compare the two values.
|
|
// FIXME: This is not correct. char + short will result in a promotion
|
|
// to int. Unfortunately we have lost types by this point.
|
|
APSIntType CompareType = std::max(APSIntType(LHSValue),
|
|
APSIntType(RHSValue));
|
|
CompareType.apply(LHSValue);
|
|
CompareType.apply(RHSValue);
|
|
} else if (!BinaryOperator::isShiftOp(op)) {
|
|
APSIntType IntType = BasicVals.getAPSIntType(resultTy);
|
|
IntType.apply(LHSValue);
|
|
IntType.apply(RHSValue);
|
|
}
|
|
|
|
std::optional<APSIntPtr> Result =
|
|
BasicVals.evalAPSInt(op, LHSValue, RHSValue);
|
|
if (!Result) {
|
|
if (op == BO_Shl || op == BO_Shr) {
|
|
// FIXME: At this point the constant folding claims that the result
|
|
// of a bitwise shift is undefined. However, constant folding
|
|
// relies on the inaccurate type information that is stored in the
|
|
// bit size of APSInt objects, and if we reached this point, then
|
|
// the checker core.BitwiseShift already determined that the shift
|
|
// is valid (in a PreStmt callback, by querying the real type from
|
|
// the AST node).
|
|
// To avoid embarrassing false positives, let's just say that we
|
|
// don't know anything about the result of the shift.
|
|
return UnknownVal();
|
|
}
|
|
return UndefinedVal();
|
|
}
|
|
|
|
return nonloc::ConcreteInt(*Result);
|
|
}
|
|
|
|
// Swap the left and right sides and flip the operator if doing so
|
|
// allows us to better reason about the expression (this is a form
|
|
// of expression canonicalization).
|
|
// While we're at it, catch some special cases for non-commutative ops.
|
|
switch (op) {
|
|
case BO_LT:
|
|
case BO_GT:
|
|
case BO_LE:
|
|
case BO_GE:
|
|
op = BinaryOperator::reverseComparisonOp(op);
|
|
[[fallthrough]];
|
|
case BO_EQ:
|
|
case BO_NE:
|
|
case BO_Add:
|
|
case BO_Mul:
|
|
case BO_And:
|
|
case BO_Xor:
|
|
case BO_Or:
|
|
std::swap(lhs, rhs);
|
|
continue;
|
|
case BO_Shr:
|
|
// (~0)>>a
|
|
if (LHSValue.isAllOnes() && LHSValue.isSigned())
|
|
return evalCast(lhs, resultTy, QualType{});
|
|
[[fallthrough]];
|
|
case BO_Shl:
|
|
// 0<<a and 0>>a
|
|
if (LHSValue == 0)
|
|
return evalCast(lhs, resultTy, QualType{});
|
|
return makeSymExprValNN(op, InputLHS, InputRHS, resultTy);
|
|
case BO_Div:
|
|
// 0 / x == 0
|
|
case BO_Rem:
|
|
// 0 % x == 0
|
|
if (LHSValue == 0)
|
|
return makeZeroVal(resultTy);
|
|
[[fallthrough]];
|
|
default:
|
|
return makeSymExprValNN(op, InputLHS, InputRHS, resultTy);
|
|
}
|
|
}
|
|
case nonloc::SymbolValKind: {
|
|
// We only handle LHS as simple symbols or SymIntExprs.
|
|
SymbolRef Sym = lhs.castAs<nonloc::SymbolVal>().getSymbol();
|
|
|
|
// LHS is a symbolic expression.
|
|
if (const SymIntExpr *symIntExpr = dyn_cast<SymIntExpr>(Sym)) {
|
|
|
|
// Is this a logical not? (!x is represented as x == 0.)
|
|
if (op == BO_EQ && rhs.isZeroConstant()) {
|
|
// We know how to negate certain expressions. Simplify them here.
|
|
|
|
BinaryOperator::Opcode opc = symIntExpr->getOpcode();
|
|
switch (opc) {
|
|
default:
|
|
// We don't know how to negate this operation.
|
|
// Just handle it as if it were a normal comparison to 0.
|
|
break;
|
|
case BO_LAnd:
|
|
case BO_LOr:
|
|
llvm_unreachable("Logical operators handled by branching logic.");
|
|
case BO_Assign:
|
|
case BO_MulAssign:
|
|
case BO_DivAssign:
|
|
case BO_RemAssign:
|
|
case BO_AddAssign:
|
|
case BO_SubAssign:
|
|
case BO_ShlAssign:
|
|
case BO_ShrAssign:
|
|
case BO_AndAssign:
|
|
case BO_XorAssign:
|
|
case BO_OrAssign:
|
|
case BO_Comma:
|
|
llvm_unreachable("'=' and ',' operators handled by ExprEngine.");
|
|
case BO_PtrMemD:
|
|
case BO_PtrMemI:
|
|
llvm_unreachable("Pointer arithmetic not handled here.");
|
|
case BO_LT:
|
|
case BO_GT:
|
|
case BO_LE:
|
|
case BO_GE:
|
|
case BO_EQ:
|
|
case BO_NE:
|
|
assert(resultTy->isBooleanType() ||
|
|
resultTy == getConditionType());
|
|
assert(symIntExpr->getType()->isBooleanType() ||
|
|
getContext().hasSameUnqualifiedType(symIntExpr->getType(),
|
|
getConditionType()));
|
|
// Negate the comparison and make a value.
|
|
opc = BinaryOperator::negateComparisonOp(opc);
|
|
return makeNonLoc(symIntExpr->getLHS(), opc,
|
|
symIntExpr->getRHS(), resultTy);
|
|
}
|
|
}
|
|
|
|
// For now, only handle expressions whose RHS is a constant.
|
|
if (const llvm::APSInt *RHSValue = getConstValue(state, rhs)) {
|
|
// If both the LHS and the current expression are additive,
|
|
// fold their constants and try again.
|
|
if (BinaryOperator::isAdditiveOp(op)) {
|
|
BinaryOperator::Opcode lop = symIntExpr->getOpcode();
|
|
if (BinaryOperator::isAdditiveOp(lop)) {
|
|
// Convert the two constants to a common type, then combine them.
|
|
|
|
// resultTy may not be the best type to convert to, but it's
|
|
// probably the best choice in expressions with mixed type
|
|
// (such as x+1U+2LL). The rules for implicit conversions should
|
|
// choose a reasonable type to preserve the expression, and will
|
|
// at least match how the value is going to be used.
|
|
APSIntType IntType = BasicVals.getAPSIntType(resultTy);
|
|
const llvm::APSInt &first = IntType.convert(symIntExpr->getRHS());
|
|
const llvm::APSInt &second = IntType.convert(*RHSValue);
|
|
|
|
// If the op and lop agrees, then we just need to
|
|
// sum the constants. Otherwise, we change to operation
|
|
// type if substraction would produce negative value
|
|
// (and cause overflow for unsigned integers),
|
|
// as consequence x+1U-10 produces x-9U, instead
|
|
// of x+4294967287U, that would be produced without this
|
|
// additional check.
|
|
std::optional<APSIntPtr> newRHS;
|
|
if (lop == op) {
|
|
newRHS = BasicVals.evalAPSInt(BO_Add, first, second);
|
|
} else if (first >= second) {
|
|
newRHS = BasicVals.evalAPSInt(BO_Sub, first, second);
|
|
op = lop;
|
|
} else {
|
|
newRHS = BasicVals.evalAPSInt(BO_Sub, second, first);
|
|
}
|
|
|
|
assert(newRHS && "Invalid operation despite common type!");
|
|
rhs = nonloc::ConcreteInt(*newRHS);
|
|
lhs = nonloc::SymbolVal(symIntExpr->getLHS());
|
|
continue;
|
|
}
|
|
}
|
|
|
|
// Otherwise, make a SymIntExpr out of the expression.
|
|
return MakeSymIntVal(symIntExpr, op, *RHSValue, resultTy);
|
|
}
|
|
}
|
|
|
|
// Is the RHS a constant?
|
|
if (const llvm::APSInt *RHSValue = getConstValue(state, rhs))
|
|
return MakeSymIntVal(Sym, op, *RHSValue, resultTy);
|
|
|
|
if (std::optional<NonLoc> V = tryRearrange(state, op, lhs, rhs, resultTy))
|
|
return *V;
|
|
|
|
// Give up -- this is not a symbolic expression we can handle.
|
|
return makeSymExprValNN(op, InputLHS, InputRHS, resultTy);
|
|
}
|
|
}
|
|
}
|
|
}
|
|
|
|
static SVal evalBinOpFieldRegionFieldRegion(const FieldRegion *LeftFR,
|
|
const FieldRegion *RightFR,
|
|
BinaryOperator::Opcode op,
|
|
QualType resultTy,
|
|
SimpleSValBuilder &SVB) {
|
|
// Only comparisons are meaningful here!
|
|
if (!BinaryOperator::isComparisonOp(op))
|
|
return UnknownVal();
|
|
|
|
// Next, see if the two FRs have the same super-region.
|
|
// FIXME: This doesn't handle casts yet, and simply stripping the casts
|
|
// doesn't help.
|
|
if (LeftFR->getSuperRegion() != RightFR->getSuperRegion())
|
|
return UnknownVal();
|
|
|
|
const FieldDecl *LeftFD = LeftFR->getDecl();
|
|
const FieldDecl *RightFD = RightFR->getDecl();
|
|
const RecordDecl *RD = LeftFD->getParent();
|
|
|
|
// Make sure the two FRs are from the same kind of record. Just in case!
|
|
// FIXME: This is probably where inheritance would be a problem.
|
|
if (RD != RightFD->getParent())
|
|
return UnknownVal();
|
|
|
|
// We know for sure that the two fields are not the same, since that
|
|
// would have given us the same SVal.
|
|
if (op == BO_EQ)
|
|
return SVB.makeTruthVal(false, resultTy);
|
|
if (op == BO_NE)
|
|
return SVB.makeTruthVal(true, resultTy);
|
|
|
|
// Iterate through the fields and see which one comes first.
|
|
// [C99 6.7.2.1.13] "Within a structure object, the non-bit-field
|
|
// members and the units in which bit-fields reside have addresses that
|
|
// increase in the order in which they are declared."
|
|
bool leftFirst = (op == BO_LT || op == BO_LE);
|
|
for (const auto *I : RD->fields()) {
|
|
if (I == LeftFD)
|
|
return SVB.makeTruthVal(leftFirst, resultTy);
|
|
if (I == RightFD)
|
|
return SVB.makeTruthVal(!leftFirst, resultTy);
|
|
}
|
|
|
|
llvm_unreachable("Fields not found in parent record's definition");
|
|
}
|
|
|
|
// This is used in debug builds only for now because some downstream users
|
|
// may hit this assert in their subsequent merges.
|
|
// There are still places in the analyzer where equal bitwidth Locs
|
|
// are compared, and need to be found and corrected. Recent previous fixes have
|
|
// addressed the known problems of making NULLs with specific bitwidths
|
|
// for Loc comparisons along with deprecation of APIs for the same purpose.
|
|
//
|
|
static void assertEqualBitWidths(ProgramStateRef State, Loc RhsLoc,
|
|
Loc LhsLoc) {
|
|
// Implements a "best effort" check for RhsLoc and LhsLoc bit widths
|
|
ASTContext &Ctx = State->getStateManager().getContext();
|
|
uint64_t RhsBitwidth =
|
|
RhsLoc.getType(Ctx).isNull() ? 0 : Ctx.getTypeSize(RhsLoc.getType(Ctx));
|
|
uint64_t LhsBitwidth =
|
|
LhsLoc.getType(Ctx).isNull() ? 0 : Ctx.getTypeSize(LhsLoc.getType(Ctx));
|
|
if (RhsBitwidth && LhsBitwidth && (LhsLoc.getKind() == RhsLoc.getKind())) {
|
|
assert(RhsBitwidth == LhsBitwidth &&
|
|
"RhsLoc and LhsLoc bitwidth must be same!");
|
|
}
|
|
}
|
|
|
|
// FIXME: all this logic will change if/when we have MemRegion::getLocation().
|
|
SVal SimpleSValBuilder::evalBinOpLL(ProgramStateRef state,
|
|
BinaryOperator::Opcode op,
|
|
Loc lhs, Loc rhs,
|
|
QualType resultTy) {
|
|
|
|
// Assert that bitwidth of lhs and rhs are the same.
|
|
// This can happen if two different address spaces are used,
|
|
// and the bitwidths of the address spaces are different.
|
|
// See LIT case clang/test/Analysis/cstring-checker-addressspace.c
|
|
// FIXME: See comment above in the function assertEqualBitWidths
|
|
assertEqualBitWidths(state, rhs, lhs);
|
|
|
|
// Only comparisons and subtractions are valid operations on two pointers.
|
|
// See [C99 6.5.5 through 6.5.14] or [C++0x 5.6 through 5.15].
|
|
// However, if a pointer is casted to an integer, evalBinOpNN may end up
|
|
// calling this function with another operation (PR7527). We don't attempt to
|
|
// model this for now, but it could be useful, particularly when the
|
|
// "location" is actually an integer value that's been passed through a void*.
|
|
if (!(BinaryOperator::isComparisonOp(op) || op == BO_Sub))
|
|
return UnknownVal();
|
|
|
|
// Special cases for when both sides are identical.
|
|
if (lhs == rhs) {
|
|
switch (op) {
|
|
default:
|
|
llvm_unreachable("Unimplemented operation for two identical values");
|
|
case BO_Sub:
|
|
return makeZeroVal(resultTy);
|
|
case BO_EQ:
|
|
case BO_LE:
|
|
case BO_GE:
|
|
return makeTruthVal(true, resultTy);
|
|
case BO_NE:
|
|
case BO_LT:
|
|
case BO_GT:
|
|
return makeTruthVal(false, resultTy);
|
|
}
|
|
}
|
|
|
|
switch (lhs.getKind()) {
|
|
default:
|
|
llvm_unreachable("Ordering not implemented for this Loc.");
|
|
|
|
case loc::GotoLabelKind:
|
|
// The only thing we know about labels is that they're non-null.
|
|
if (rhs.isZeroConstant()) {
|
|
switch (op) {
|
|
default:
|
|
break;
|
|
case BO_Sub:
|
|
return evalCast(lhs, resultTy, QualType{});
|
|
case BO_EQ:
|
|
case BO_LE:
|
|
case BO_LT:
|
|
return makeTruthVal(false, resultTy);
|
|
case BO_NE:
|
|
case BO_GT:
|
|
case BO_GE:
|
|
return makeTruthVal(true, resultTy);
|
|
}
|
|
}
|
|
// There may be two labels for the same location, and a function region may
|
|
// have the same address as a label at the start of the function (depending
|
|
// on the ABI).
|
|
// FIXME: we can probably do a comparison against other MemRegions, though.
|
|
// FIXME: is there a way to tell if two labels refer to the same location?
|
|
return UnknownVal();
|
|
|
|
case loc::ConcreteIntKind: {
|
|
auto L = lhs.castAs<loc::ConcreteInt>();
|
|
|
|
// If one of the operands is a symbol and the other is a constant,
|
|
// build an expression for use by the constraint manager.
|
|
if (SymbolRef rSym = rhs.getAsLocSymbol()) {
|
|
if (op == BO_Cmp)
|
|
return UnknownVal();
|
|
|
|
if (!BinaryOperator::isComparisonOp(op))
|
|
return makeNonLoc(L.getValue(), op, rSym, resultTy);
|
|
|
|
op = BinaryOperator::reverseComparisonOp(op);
|
|
return makeNonLoc(rSym, op, L.getValue(), resultTy);
|
|
}
|
|
|
|
// If both operands are constants, just perform the operation.
|
|
if (std::optional<loc::ConcreteInt> rInt = rhs.getAs<loc::ConcreteInt>()) {
|
|
assert(BinaryOperator::isComparisonOp(op) || op == BO_Sub);
|
|
|
|
if (std::optional<APSIntPtr> ResultInt =
|
|
BasicVals.evalAPSInt(op, L.getValue(), rInt->getValue()))
|
|
return evalCast(nonloc::ConcreteInt(*ResultInt), resultTy, QualType{});
|
|
return UnknownVal();
|
|
}
|
|
|
|
// Special case comparisons against NULL.
|
|
// This must come after the test if the RHS is a symbol, which is used to
|
|
// build constraints. The address of any non-symbolic region is guaranteed
|
|
// to be non-NULL, as is any label.
|
|
assert((isa<loc::MemRegionVal, loc::GotoLabel>(rhs)));
|
|
if (lhs.isZeroConstant()) {
|
|
switch (op) {
|
|
default:
|
|
break;
|
|
case BO_EQ:
|
|
case BO_GT:
|
|
case BO_GE:
|
|
return makeTruthVal(false, resultTy);
|
|
case BO_NE:
|
|
case BO_LT:
|
|
case BO_LE:
|
|
return makeTruthVal(true, resultTy);
|
|
}
|
|
}
|
|
|
|
// Comparing an arbitrary integer to a region or label address is
|
|
// completely unknowable.
|
|
return UnknownVal();
|
|
}
|
|
case loc::MemRegionValKind: {
|
|
if (std::optional<loc::ConcreteInt> rInt = rhs.getAs<loc::ConcreteInt>()) {
|
|
// If one of the operands is a symbol and the other is a constant,
|
|
// build an expression for use by the constraint manager.
|
|
if (SymbolRef lSym = lhs.getAsLocSymbol(true)) {
|
|
if (BinaryOperator::isComparisonOp(op))
|
|
return MakeSymIntVal(lSym, op, rInt->getValue(), resultTy);
|
|
return UnknownVal();
|
|
}
|
|
// Special case comparisons to NULL.
|
|
// This must come after the test if the LHS is a symbol, which is used to
|
|
// build constraints. The address of any non-symbolic region is guaranteed
|
|
// to be non-NULL.
|
|
if (rInt->isZeroConstant()) {
|
|
if (op == BO_Sub)
|
|
return evalCast(lhs, resultTy, QualType{});
|
|
|
|
if (BinaryOperator::isComparisonOp(op)) {
|
|
QualType boolType = getContext().BoolTy;
|
|
NonLoc l = evalCast(lhs, boolType, QualType{}).castAs<NonLoc>();
|
|
NonLoc r = makeTruthVal(false, boolType).castAs<NonLoc>();
|
|
return evalBinOpNN(state, op, l, r, resultTy);
|
|
}
|
|
}
|
|
|
|
// Comparing a region to an arbitrary integer is completely unknowable.
|
|
return UnknownVal();
|
|
}
|
|
|
|
// Get both values as regions, if possible.
|
|
const MemRegion *LeftMR = lhs.getAsRegion();
|
|
assert(LeftMR && "MemRegionValKind SVal doesn't have a region!");
|
|
|
|
const MemRegion *RightMR = rhs.getAsRegion();
|
|
if (!RightMR)
|
|
// The RHS is probably a label, which in theory could address a region.
|
|
// FIXME: we can probably make a more useful statement about non-code
|
|
// regions, though.
|
|
return UnknownVal();
|
|
|
|
const MemRegion *LeftBase = LeftMR->getBaseRegion();
|
|
const MemRegion *RightBase = RightMR->getBaseRegion();
|
|
const MemSpaceRegion *LeftMS = LeftBase->getMemorySpace(state);
|
|
const MemSpaceRegion *RightMS = RightBase->getMemorySpace(state);
|
|
const MemSpaceRegion *UnknownMS = MemMgr.getUnknownRegion();
|
|
|
|
// If the two regions are from different known memory spaces they cannot be
|
|
// equal. Also, assume that no symbolic region (whose memory space is
|
|
// unknown) is on the stack.
|
|
if (LeftMS != RightMS &&
|
|
((LeftMS != UnknownMS && RightMS != UnknownMS) ||
|
|
(isa<StackSpaceRegion>(LeftMS) || isa<StackSpaceRegion>(RightMS)))) {
|
|
switch (op) {
|
|
default:
|
|
return UnknownVal();
|
|
case BO_EQ:
|
|
return makeTruthVal(false, resultTy);
|
|
case BO_NE:
|
|
return makeTruthVal(true, resultTy);
|
|
}
|
|
}
|
|
|
|
// If both values wrap regions, see if they're from different base regions.
|
|
// Note, heap base symbolic regions are assumed to not alias with
|
|
// each other; for example, we assume that malloc returns different address
|
|
// on each invocation.
|
|
// FIXME: ObjC object pointers always reside on the heap, but currently
|
|
// we treat their memory space as unknown, because symbolic pointers
|
|
// to ObjC objects may alias. There should be a way to construct
|
|
// possibly-aliasing heap-based regions. For instance, MacOSXApiChecker
|
|
// guesses memory space for ObjC object pointers manually instead of
|
|
// relying on us.
|
|
if (LeftBase != RightBase &&
|
|
((!isa<SymbolicRegion>(LeftBase) && !isa<SymbolicRegion>(RightBase)) ||
|
|
(isa<HeapSpaceRegion>(LeftMS) || isa<HeapSpaceRegion>(RightMS))) ){
|
|
switch (op) {
|
|
default:
|
|
return UnknownVal();
|
|
case BO_EQ:
|
|
return makeTruthVal(false, resultTy);
|
|
case BO_NE:
|
|
return makeTruthVal(true, resultTy);
|
|
}
|
|
}
|
|
|
|
// Handle special cases for when both regions are element regions.
|
|
const ElementRegion *RightER = dyn_cast<ElementRegion>(RightMR);
|
|
const ElementRegion *LeftER = dyn_cast<ElementRegion>(LeftMR);
|
|
if (RightER && LeftER) {
|
|
// Next, see if the two ERs have the same super-region and matching types.
|
|
// FIXME: This should do something useful even if the types don't match,
|
|
// though if both indexes are constant the RegionRawOffset path will
|
|
// give the correct answer.
|
|
if (LeftER->getSuperRegion() == RightER->getSuperRegion() &&
|
|
LeftER->getElementType() == RightER->getElementType()) {
|
|
// Get the left index and cast it to the correct type.
|
|
// If the index is unknown or undefined, bail out here.
|
|
SVal LeftIndexVal = LeftER->getIndex();
|
|
std::optional<NonLoc> LeftIndex = LeftIndexVal.getAs<NonLoc>();
|
|
if (!LeftIndex)
|
|
return UnknownVal();
|
|
LeftIndexVal = evalCast(*LeftIndex, ArrayIndexTy, QualType{});
|
|
LeftIndex = LeftIndexVal.getAs<NonLoc>();
|
|
if (!LeftIndex)
|
|
return UnknownVal();
|
|
|
|
// Do the same for the right index.
|
|
SVal RightIndexVal = RightER->getIndex();
|
|
std::optional<NonLoc> RightIndex = RightIndexVal.getAs<NonLoc>();
|
|
if (!RightIndex)
|
|
return UnknownVal();
|
|
RightIndexVal = evalCast(*RightIndex, ArrayIndexTy, QualType{});
|
|
RightIndex = RightIndexVal.getAs<NonLoc>();
|
|
if (!RightIndex)
|
|
return UnknownVal();
|
|
|
|
// Actually perform the operation.
|
|
// evalBinOpNN expects the two indexes to already be the right type.
|
|
return evalBinOpNN(state, op, *LeftIndex, *RightIndex, resultTy);
|
|
}
|
|
}
|
|
|
|
// Special handling of the FieldRegions, even with symbolic offsets.
|
|
const FieldRegion *RightFR = dyn_cast<FieldRegion>(RightMR);
|
|
const FieldRegion *LeftFR = dyn_cast<FieldRegion>(LeftMR);
|
|
if (RightFR && LeftFR) {
|
|
SVal R = evalBinOpFieldRegionFieldRegion(LeftFR, RightFR, op, resultTy,
|
|
*this);
|
|
if (!R.isUnknown())
|
|
return R;
|
|
}
|
|
|
|
// Compare the regions using the raw offsets.
|
|
RegionOffset LeftOffset = LeftMR->getAsOffset();
|
|
RegionOffset RightOffset = RightMR->getAsOffset();
|
|
|
|
if (LeftOffset.getRegion() != nullptr &&
|
|
LeftOffset.getRegion() == RightOffset.getRegion() &&
|
|
!LeftOffset.hasSymbolicOffset() && !RightOffset.hasSymbolicOffset()) {
|
|
int64_t left = LeftOffset.getOffset();
|
|
int64_t right = RightOffset.getOffset();
|
|
|
|
switch (op) {
|
|
default:
|
|
return UnknownVal();
|
|
case BO_LT:
|
|
return makeTruthVal(left < right, resultTy);
|
|
case BO_GT:
|
|
return makeTruthVal(left > right, resultTy);
|
|
case BO_LE:
|
|
return makeTruthVal(left <= right, resultTy);
|
|
case BO_GE:
|
|
return makeTruthVal(left >= right, resultTy);
|
|
case BO_EQ:
|
|
return makeTruthVal(left == right, resultTy);
|
|
case BO_NE:
|
|
return makeTruthVal(left != right, resultTy);
|
|
}
|
|
}
|
|
|
|
// At this point we're not going to get a good answer, but we can try
|
|
// conjuring an expression instead.
|
|
SymbolRef LHSSym = lhs.getAsLocSymbol();
|
|
SymbolRef RHSSym = rhs.getAsLocSymbol();
|
|
if (LHSSym && RHSSym)
|
|
return makeNonLoc(LHSSym, op, RHSSym, resultTy);
|
|
|
|
// If we get here, we have no way of comparing the regions.
|
|
return UnknownVal();
|
|
}
|
|
}
|
|
}
|
|
|
|
SVal SimpleSValBuilder::evalBinOpLN(ProgramStateRef state,
|
|
BinaryOperator::Opcode op, Loc lhs,
|
|
NonLoc rhs, QualType resultTy) {
|
|
if (op >= BO_PtrMemD && op <= BO_PtrMemI) {
|
|
if (auto PTMSV = rhs.getAs<nonloc::PointerToMember>()) {
|
|
if (PTMSV->isNullMemberPointer())
|
|
return UndefinedVal();
|
|
|
|
auto getFieldLValue = [&](const auto *FD) -> SVal {
|
|
SVal Result = lhs;
|
|
|
|
for (const auto &I : *PTMSV)
|
|
Result = StateMgr.getStoreManager().evalDerivedToBase(
|
|
Result, I->getType(), I->isVirtual());
|
|
|
|
return state->getLValue(FD, Result);
|
|
};
|
|
|
|
if (const auto *FD = PTMSV->getDeclAs<FieldDecl>()) {
|
|
return getFieldLValue(FD);
|
|
}
|
|
if (const auto *FD = PTMSV->getDeclAs<IndirectFieldDecl>()) {
|
|
return getFieldLValue(FD);
|
|
}
|
|
}
|
|
|
|
return rhs;
|
|
}
|
|
|
|
assert(!BinaryOperator::isComparisonOp(op) &&
|
|
"arguments to comparison ops must be of the same type");
|
|
|
|
// Special case: rhs is a zero constant.
|
|
if (rhs.isZeroConstant())
|
|
return lhs;
|
|
|
|
// Perserve the null pointer so that it can be found by the DerefChecker.
|
|
if (lhs.isZeroConstant())
|
|
return lhs;
|
|
|
|
// We are dealing with pointer arithmetic.
|
|
|
|
// Handle pointer arithmetic on constant values.
|
|
if (std::optional<nonloc::ConcreteInt> rhsInt =
|
|
rhs.getAs<nonloc::ConcreteInt>()) {
|
|
if (std::optional<loc::ConcreteInt> lhsInt =
|
|
lhs.getAs<loc::ConcreteInt>()) {
|
|
const llvm::APSInt &leftI = lhsInt->getValue();
|
|
assert(leftI.isUnsigned());
|
|
llvm::APSInt rightI(rhsInt->getValue(), /* isUnsigned */ true);
|
|
|
|
// Convert the bitwidth of rightI. This should deal with overflow
|
|
// since we are dealing with concrete values.
|
|
rightI = rightI.extOrTrunc(leftI.getBitWidth());
|
|
|
|
// Offset the increment by the pointer size.
|
|
llvm::APSInt Multiplicand(rightI.getBitWidth(), /* isUnsigned */ true);
|
|
QualType pointeeType = resultTy->getPointeeType();
|
|
Multiplicand = getContext().getTypeSizeInChars(pointeeType).getQuantity();
|
|
rightI *= Multiplicand;
|
|
|
|
// Compute the adjusted pointer.
|
|
switch (op) {
|
|
case BO_Add:
|
|
rightI = leftI + rightI;
|
|
break;
|
|
case BO_Sub:
|
|
rightI = leftI - rightI;
|
|
break;
|
|
default:
|
|
llvm_unreachable("Invalid pointer arithmetic operation");
|
|
}
|
|
return loc::ConcreteInt(getBasicValueFactory().getValue(rightI));
|
|
}
|
|
}
|
|
|
|
// Handle cases where 'lhs' is a region.
|
|
if (const MemRegion *region = lhs.getAsRegion()) {
|
|
rhs = convertToArrayIndex(rhs).castAs<NonLoc>();
|
|
SVal index = UnknownVal();
|
|
const SubRegion *superR = nullptr;
|
|
// We need to know the type of the pointer in order to add an integer to it.
|
|
// Depending on the type, different amount of bytes is added.
|
|
QualType elementType;
|
|
|
|
if (const ElementRegion *elemReg = dyn_cast<ElementRegion>(region)) {
|
|
assert(op == BO_Add || op == BO_Sub);
|
|
index = evalBinOpNN(state, op, elemReg->getIndex(), rhs,
|
|
getArrayIndexType());
|
|
superR = cast<SubRegion>(elemReg->getSuperRegion());
|
|
elementType = elemReg->getElementType();
|
|
}
|
|
else if (isa<SubRegion>(region)) {
|
|
assert(op == BO_Add || op == BO_Sub);
|
|
index = (op == BO_Add) ? rhs : evalMinus(rhs);
|
|
superR = cast<SubRegion>(region);
|
|
// TODO: Is this actually reliable? Maybe improving our MemRegion
|
|
// hierarchy to provide typed regions for all non-void pointers would be
|
|
// better. For instance, we cannot extend this towards LocAsInteger
|
|
// operations, where result type of the expression is integer.
|
|
if (resultTy->isAnyPointerType())
|
|
elementType = resultTy->getPointeeType();
|
|
}
|
|
|
|
// Represent arithmetic on void pointers as arithmetic on char pointers.
|
|
// It is fine when a TypedValueRegion of char value type represents
|
|
// a void pointer. Note that arithmetic on void pointers is a GCC extension.
|
|
if (elementType->isVoidType())
|
|
elementType = getContext().CharTy;
|
|
|
|
if (std::optional<NonLoc> indexV = index.getAs<NonLoc>()) {
|
|
return loc::MemRegionVal(MemMgr.getElementRegion(elementType, *indexV,
|
|
superR, getContext()));
|
|
}
|
|
}
|
|
return UnknownVal();
|
|
}
|
|
|
|
const llvm::APSInt *SimpleSValBuilder::getConstValue(ProgramStateRef state,
|
|
SVal V) {
|
|
if (const llvm::APSInt *Res = getConcreteValue(V))
|
|
return Res;
|
|
|
|
if (SymbolRef Sym = V.getAsSymbol())
|
|
return state->getConstraintManager().getSymVal(state, Sym);
|
|
|
|
return nullptr;
|
|
}
|
|
|
|
const llvm::APSInt *SimpleSValBuilder::getConcreteValue(SVal V) {
|
|
if (std::optional<loc::ConcreteInt> X = V.getAs<loc::ConcreteInt>())
|
|
return X->getValue().get();
|
|
|
|
if (std::optional<nonloc::ConcreteInt> X = V.getAs<nonloc::ConcreteInt>())
|
|
return X->getValue().get();
|
|
|
|
return nullptr;
|
|
}
|
|
|
|
const llvm::APSInt *SimpleSValBuilder::getKnownValue(ProgramStateRef state,
|
|
SVal V) {
|
|
return getConstValue(state, simplifySVal(state, V));
|
|
}
|
|
|
|
const llvm::APSInt *SimpleSValBuilder::getMinValue(ProgramStateRef state,
|
|
SVal V) {
|
|
V = simplifySVal(state, V);
|
|
|
|
if (const llvm::APSInt *Res = getConcreteValue(V))
|
|
return Res;
|
|
|
|
if (SymbolRef Sym = V.getAsSymbol())
|
|
return state->getConstraintManager().getSymMinVal(state, Sym);
|
|
|
|
return nullptr;
|
|
}
|
|
|
|
const llvm::APSInt *SimpleSValBuilder::getMaxValue(ProgramStateRef state,
|
|
SVal V) {
|
|
V = simplifySVal(state, V);
|
|
|
|
if (const llvm::APSInt *Res = getConcreteValue(V))
|
|
return Res;
|
|
|
|
if (SymbolRef Sym = V.getAsSymbol())
|
|
return state->getConstraintManager().getSymMaxVal(state, Sym);
|
|
|
|
return nullptr;
|
|
}
|
|
|
|
SVal SimpleSValBuilder::simplifyUntilFixpoint(ProgramStateRef State, SVal Val) {
|
|
SVal SimplifiedVal = simplifySValOnce(State, Val);
|
|
while (SimplifiedVal != Val) {
|
|
Val = SimplifiedVal;
|
|
SimplifiedVal = simplifySValOnce(State, Val);
|
|
}
|
|
return SimplifiedVal;
|
|
}
|
|
|
|
SVal SimpleSValBuilder::simplifySVal(ProgramStateRef State, SVal V) {
|
|
return simplifyUntilFixpoint(State, V);
|
|
}
|
|
|
|
SVal SimpleSValBuilder::simplifySValOnce(ProgramStateRef State, SVal V) {
|
|
// For now, this function tries to constant-fold symbols inside a
|
|
// nonloc::SymbolVal, and does nothing else. More simplifications should
|
|
// be possible, such as constant-folding an index in an ElementRegion.
|
|
|
|
class Simplifier : public FullSValVisitor<Simplifier, SVal> {
|
|
ProgramStateRef State;
|
|
SValBuilder &SVB;
|
|
|
|
// Cache results for the lifetime of the Simplifier. Results change every
|
|
// time new constraints are added to the program state, which is the whole
|
|
// point of simplifying, and for that very reason it's pointless to maintain
|
|
// the same cache for the duration of the whole analysis.
|
|
llvm::DenseMap<SymbolRef, SVal> Cached;
|
|
|
|
static bool isUnchanged(SymbolRef Sym, SVal Val) {
|
|
return Sym == Val.getAsSymbol();
|
|
}
|
|
|
|
SVal cache(SymbolRef Sym, SVal V) {
|
|
Cached[Sym] = V;
|
|
return V;
|
|
}
|
|
|
|
SVal skip(SymbolRef Sym) {
|
|
return cache(Sym, SVB.makeSymbolVal(Sym));
|
|
}
|
|
|
|
// Return the known const value for the Sym if available, or return Undef
|
|
// otherwise.
|
|
SVal getConst(SymbolRef Sym) {
|
|
const llvm::APSInt *Const =
|
|
State->getConstraintManager().getSymVal(State, Sym);
|
|
if (Const)
|
|
return Loc::isLocType(Sym->getType()) ? (SVal)SVB.makeIntLocVal(*Const)
|
|
: (SVal)SVB.makeIntVal(*Const);
|
|
return UndefinedVal();
|
|
}
|
|
|
|
SVal getConstOrVisit(SymbolRef Sym) {
|
|
const SVal Ret = getConst(Sym);
|
|
if (Ret.isUndef())
|
|
return Visit(Sym);
|
|
return Ret;
|
|
}
|
|
|
|
public:
|
|
Simplifier(ProgramStateRef State)
|
|
: State(State), SVB(State->getStateManager().getSValBuilder()) {}
|
|
|
|
SVal VisitSymbolData(const SymbolData *S) {
|
|
// No cache here.
|
|
if (const llvm::APSInt *I =
|
|
State->getConstraintManager().getSymVal(State, S))
|
|
return Loc::isLocType(S->getType()) ? (SVal)SVB.makeIntLocVal(*I)
|
|
: (SVal)SVB.makeIntVal(*I);
|
|
return SVB.makeSymbolVal(S);
|
|
}
|
|
|
|
SVal VisitSymIntExpr(const SymIntExpr *S) {
|
|
auto I = Cached.find(S);
|
|
if (I != Cached.end())
|
|
return I->second;
|
|
|
|
SVal LHS = getConstOrVisit(S->getLHS());
|
|
if (isUnchanged(S->getLHS(), LHS))
|
|
return skip(S);
|
|
|
|
SVal RHS;
|
|
// By looking at the APSInt in the right-hand side of S, we cannot
|
|
// figure out if it should be treated as a Loc or as a NonLoc.
|
|
// So make our guess by recalling that we cannot multiply pointers
|
|
// or compare a pointer to an integer.
|
|
if (Loc::isLocType(S->getLHS()->getType()) &&
|
|
BinaryOperator::isComparisonOp(S->getOpcode())) {
|
|
// The usual conversion of $sym to &SymRegion{$sym}, as they have
|
|
// the same meaning for Loc-type symbols, but the latter form
|
|
// is preferred in SVal computations for being Loc itself.
|
|
if (SymbolRef Sym = LHS.getAsSymbol()) {
|
|
assert(Loc::isLocType(Sym->getType()));
|
|
LHS = SVB.makeLoc(Sym);
|
|
}
|
|
RHS = SVB.makeIntLocVal(S->getRHS());
|
|
} else {
|
|
RHS = SVB.makeIntVal(S->getRHS());
|
|
}
|
|
|
|
return cache(
|
|
S, SVB.evalBinOp(State, S->getOpcode(), LHS, RHS, S->getType()));
|
|
}
|
|
|
|
SVal VisitIntSymExpr(const IntSymExpr *S) {
|
|
auto I = Cached.find(S);
|
|
if (I != Cached.end())
|
|
return I->second;
|
|
|
|
SVal RHS = getConstOrVisit(S->getRHS());
|
|
if (isUnchanged(S->getRHS(), RHS))
|
|
return skip(S);
|
|
|
|
SVal LHS = SVB.makeIntVal(S->getLHS());
|
|
return cache(
|
|
S, SVB.evalBinOp(State, S->getOpcode(), LHS, RHS, S->getType()));
|
|
}
|
|
|
|
SVal VisitSymSymExpr(const SymSymExpr *S) {
|
|
auto I = Cached.find(S);
|
|
if (I != Cached.end())
|
|
return I->second;
|
|
|
|
// For now don't try to simplify mixed Loc/NonLoc expressions
|
|
// because they often appear from LocAsInteger operations
|
|
// and we don't know how to combine a LocAsInteger
|
|
// with a concrete value.
|
|
if (Loc::isLocType(S->getLHS()->getType()) !=
|
|
Loc::isLocType(S->getRHS()->getType()))
|
|
return skip(S);
|
|
|
|
SVal LHS = getConstOrVisit(S->getLHS());
|
|
SVal RHS = getConstOrVisit(S->getRHS());
|
|
|
|
if (isUnchanged(S->getLHS(), LHS) && isUnchanged(S->getRHS(), RHS))
|
|
return skip(S);
|
|
|
|
return cache(
|
|
S, SVB.evalBinOp(State, S->getOpcode(), LHS, RHS, S->getType()));
|
|
}
|
|
|
|
SVal VisitSymbolCast(const SymbolCast *S) {
|
|
auto I = Cached.find(S);
|
|
if (I != Cached.end())
|
|
return I->second;
|
|
const SymExpr *OpSym = S->getOperand();
|
|
SVal OpVal = getConstOrVisit(OpSym);
|
|
if (isUnchanged(OpSym, OpVal))
|
|
return skip(S);
|
|
|
|
return cache(S, SVB.evalCast(OpVal, S->getType(), OpSym->getType()));
|
|
}
|
|
|
|
SVal VisitUnarySymExpr(const UnarySymExpr *S) {
|
|
auto I = Cached.find(S);
|
|
if (I != Cached.end())
|
|
return I->second;
|
|
SVal Op = getConstOrVisit(S->getOperand());
|
|
if (isUnchanged(S->getOperand(), Op))
|
|
return skip(S);
|
|
|
|
return cache(
|
|
S, SVB.evalUnaryOp(State, S->getOpcode(), Op, S->getType()));
|
|
}
|
|
|
|
SVal VisitSymExpr(SymbolRef S) { return nonloc::SymbolVal(S); }
|
|
|
|
SVal VisitMemRegion(const MemRegion *R) { return loc::MemRegionVal(R); }
|
|
|
|
SVal VisitSymbolVal(nonloc::SymbolVal V) {
|
|
// Simplification is much more costly than computing complexity.
|
|
// For high complexity, it may be not worth it.
|
|
return Visit(V.getSymbol());
|
|
}
|
|
|
|
SVal VisitSVal(SVal V) { return V; }
|
|
};
|
|
|
|
SVal SimplifiedV = Simplifier(State).Visit(V);
|
|
return SimplifiedV;
|
|
}
|